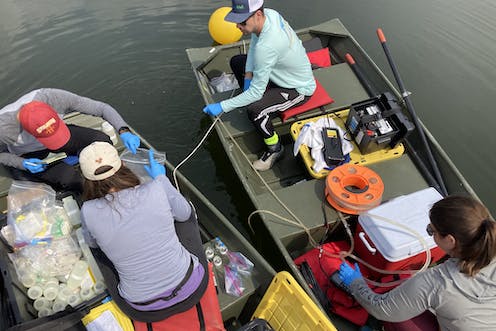
Researchers sample water from various layers to analyze back in the lab. Elizabeth Swanner, CC BY-ND
Little Deming Lake doesn’t get much notice from visitors to Itasca State Park in Minnesota. There’s better boating on nearby Lake Itasca, the headwaters of the Mississippi River. My colleagues and I need to maneuver hundreds of pounds of equipment down a hidden path made narrow by late-summer poison ivy to launch our rowboats.
But modest Deming Lake offers more than meets the eye for me, a geochemist interested in how oxygen built up in the atmosphere 2.4 billion years ago. The absence of oxygen in the deep layers of Deming Lake is something this small body of water has in common with early Earth’s oceans.
On each of our several expeditions here each year, we row our boats out into the deepest part of the lake – over 60 feet (18 meters), despite the lake’s surface area being only 13 acres. We drop an anchor and connect our boats in a flotilla, readying ourselves for the work ahead.
Researchers’ boats on Deming Lake.
Elizabeth Swanner, CC BY-ND
Deming Lake is meromictic, a term from Greek that means only partially mixing. In most lakes, at least once a year, the water at the top sinks while the water at the bottom rises because of wind and seasonal temperature changes that affect water’s density. But the deepest waters of Deming Lake never reach the surface. This prevents oxygen in its top layer of water from ever mixing into its deep layer.
Less than 1% of lakes are meromictic, and most that are have dense, salty bottom waters. Deming Lake’s deep waters are not very salty, but of the salts in its bottom waters, iron is one of the most abundant. This makes Deming Lake one of the rarest types of meromictic lakes.
Postdoc researcher Sajjad Akam collects a water sample for chemical analysis back in the lab.
Elizabeth Swanner, CC BY-ND
The lake surface is calm, and the still air is glorious on this cool, cloudless August morning. We lower a 2-foot-long water pump zip-tied to a cable attached to four sensors. The sensors measure the temperature, amount of oxygen, pH and amount of chlorophyll in the water at each layer we encounter. We pump water from the most intriguing layers up to the boat and fill a myriad of bottles and tubes, each destined for a different chemical or biological analysis.
My colleagues and I have homed in on Deming Lake to explore questions about how microbial life adapted to and changed the environmental conditions on early Earth. Our planet was inhabited only by microbes for most of its history. The atmosphere and the oceans’ depths didn’t have much oxygen, but they did have a lot of iron, just like Deming Lake does. By investigating what Deming Lake’s microbes are doing, we can better understand how billions of years ago they helped to transform the Earth’s atmosphere and oceans into what they’re like now.
Layer by layer, into the lake
Two and a half billion years ago, ocean waters had enough iron to form today’s globally distributed rusty iron deposits called banded iron formations that supply iron for the modern global steel industry. Nowadays, oceans have only trace amounts of iron but abundant oxygen. In most waters, iron and oxygen are antithetical. Rapid chemical and biological reactions between iron and oxygen mean you can’t have much of one while the other is present.
The rise of oxygen in the early atmosphere and ocean was due to cyanobacteria. These single-celled organisms emerged at least 2.5 billion years ago. But it took roughly 2 billion years for the oxygen they produce via photosynthesis to build up to levels that allowed for the first animals to appear on Earth.
Chlorophyll colors water from the lake slightly green.
Elizabeth Swanner, CC BY-ND
At Deming Lake, my colleagues and I pay special attention to the water layer where the chlorophyll readings jump. Chlorophyll is the pigment that makes plants green. It harnesses sunlight energy to turn water and carbon dioxide into oxygen and sugars. Nearly 20 feet (6 meters) below Deming’s surface, the chlorophyll is in cyanobacteria and photosynthetic algae, not plants.
But the curious thing about this layer is that we don’t detect oxygen, despite the abundance of these oxygen-producing organisms. This is the depth where iron concentrations start to climb to the high levels present at the lake’s bottom.
This high-chlorophyll, high-iron and low-oxygen layer is of special interest to us because it might help us understand where cyanobacteria lived in the ancient ocean, how well they were growing and how much oxygen they produced.
We suspect the reason cyanobacteria gather at this depth in Deming Lake is that there is more iron there than at the top of the lake. Just like humans need iron for red blood cells, cyanobacteria need lots of iron to help catalyze the reactions of photosynthesis.
A likely reason we can’t measure any oxygen in this layer is that in addition to cyanobacteria, there are a lot of other bacteria here. After a good long life of a few days, the cyanobacteria die, and the other bacteria feed on their remains. These bacteria rapidly use up any oxygen produced by still photosynthesizing cyanobacteria the way a fire does as it burns through wood.
We know there are lots of bacteria here based on how cloudy the water is, and we see them when we inspect a drop of this water under a microscope. But we need another way to measure photosynthesis besides measuring oxygen levels.
Long-running lakeside laboratory
The other important function of photosynthesis is converting carbon dioxide into sugars, which eventually are used to make more cells. We need a way to track whether new sugars are being made, and if they are, whether it’s by photosynthetic cyanobacteria. So we fill glass bottles with samples of water from this lake layer and seal them tight with rubber stoppers.
We drive the 3 miles back to the Itasca Biological Station and Laboratories where we will set up our experiments. The station opened in 1909 and is home base for us this week, providing comfy cabins, warm meals and this laboratory space.
In the lab, we inject our glass bottle with carbon dioxide that carries an isotopic tracer. If cyanobacteria grow, their cells will incorporate this isotopic marker.
We had a little help to formulate our questions and experiments. University of Minnesota students attending summer field courses collected decades worth of data in Itasca State Park. A diligent university librarian digitized thousands of those students’ final papers.
My students and I pored over the papers concerning Deming Lake, many of which tried to determine whether the cyanobacteria in the chlorophyll-rich layer are doing photosynthesis. While most indicated yes, those students were measuring only oxygen and got ambiguous results. Our use of the isotopic tracer is trickier to implement but will give clearer results.
Graduate students Michelle Chamberlain and Zackry Stevenson about to sink the bottles for incubation in Deming Lake.
Elizabeth Swanner, CC BY-ND
That afternoon, we’re back on the lake. We toss an anchor; attached to its rope is a clear plastic bag holding the sealed bottles of lake water now amended with the isotopic tracer. They’ll spend the night in the chlorophyll-rich layer, and we’ll retrieve them after 24 hours. Any longer than that and the isotopic label might end up in the bacteria that eat the dying cyanobacteria instead of the cyanobacteria themselves. We tie off the rope to a floating buoy and head back to the station’s dining hall for our evening meal.
Iron, chlorophyll, oxygen
The next morning, as we wait for the bottles to finish their incubation, we collect water from the different layers of the lake and add some chemicals that kill the cells but preserve their bodies. We’ll look at these samples under the microscope to figure out how many cyanobacteria are in the water, and we’ll measure how much iron is inside the cyanobacteria.
That’s easier said than done, because we have to first separate all the “needles” (cyanobacteria) from the “hay” (other cells) and then clean any iron off the outside of the cyanobacteria. Back at Iowa State University, we’ll shoot the individual cells one by one into a flame that incinerates them, which liberates all the iron they contain so we can measure it.
Biogeochemist Katy Sparrow rows a research vessel to shore.
Elizabeth Swanner, CC BY-ND
Our scientific hunch, or hypothesis, is that the cyanobacteria that live in the chlorophyll- and iron-rich layer will contain more iron than cyanobacteria that live in the top lake layer. If they do, it will help us establish that greater access to iron is a motive for living in that deeper and dimmer layer.
These experiments won’t tell the whole story of why it took so long for Earth to build up oxygen, but they will help us to understand a piece of it – where oxygen might have been produced and why, and what happened to oxygen in that environment.
Deming Lake is quickly becoming its own attraction for those with a curiosity about what goes on beneath its tranquil surface – and what that might be able to tell us about how new forms of life took hold long ago on Earth.
Elizabeth Swanner receives funding from the U.S. National Science Foundation and the National Aeronautics and Space Administration.
Advertisement

Advertisement
Contact Us
If you would like to place dofollow backlinks in our website or paid content reach out to info@qhubonews.com